Sodium Manganese Oxid – IBUvolt® NMO
Cathode Material in Sodium-Ion Batteries
IBUvolt® NMO is a newly developed cathode material for use in modern batteries. Due to its non-hazardous and non-toxic ingredients, NMO (sodium manganese oxide, NaxMnO2) is considered a particularly safe battery material and is already being used in electromobility and stationary energy storage systems.
We have been active in the field of cathode production for lithium-ion batteries for over 10 years. We have now used our extensive experience in product and process development in combination with our expertise in thermal process engineering to develop a cathode material for sodium-ion batteries. The sodium-manganese layered oxide IBUvolt® NMO represents the beginning of this development and offers a ready-to-use cathode material thanks to its high purity and the industrially continuous production process.
Readily available and cost-effective raw material
No critical elements such as lithium, cobalt and nickel
High cycle stability
Scalable, continuous production process: efficient synthesis with fewer by-products and waste products, energy-efficient production process
Phase-pure material
Sodium Manganese Oxide – Production and Process Description
Our team has been working for some time on the development and optimization of NMO from laboratory to industrial scale, funded by the BMBF (03XP0404A). The company's expertise in thermal process engineering is used for production and a two-stage process has been developed in which the cathode precursor compound is first produced in a pulsation reactor and then refined in a rotary kiln. This makes the process not only unique, but also comparatively energy-efficient.
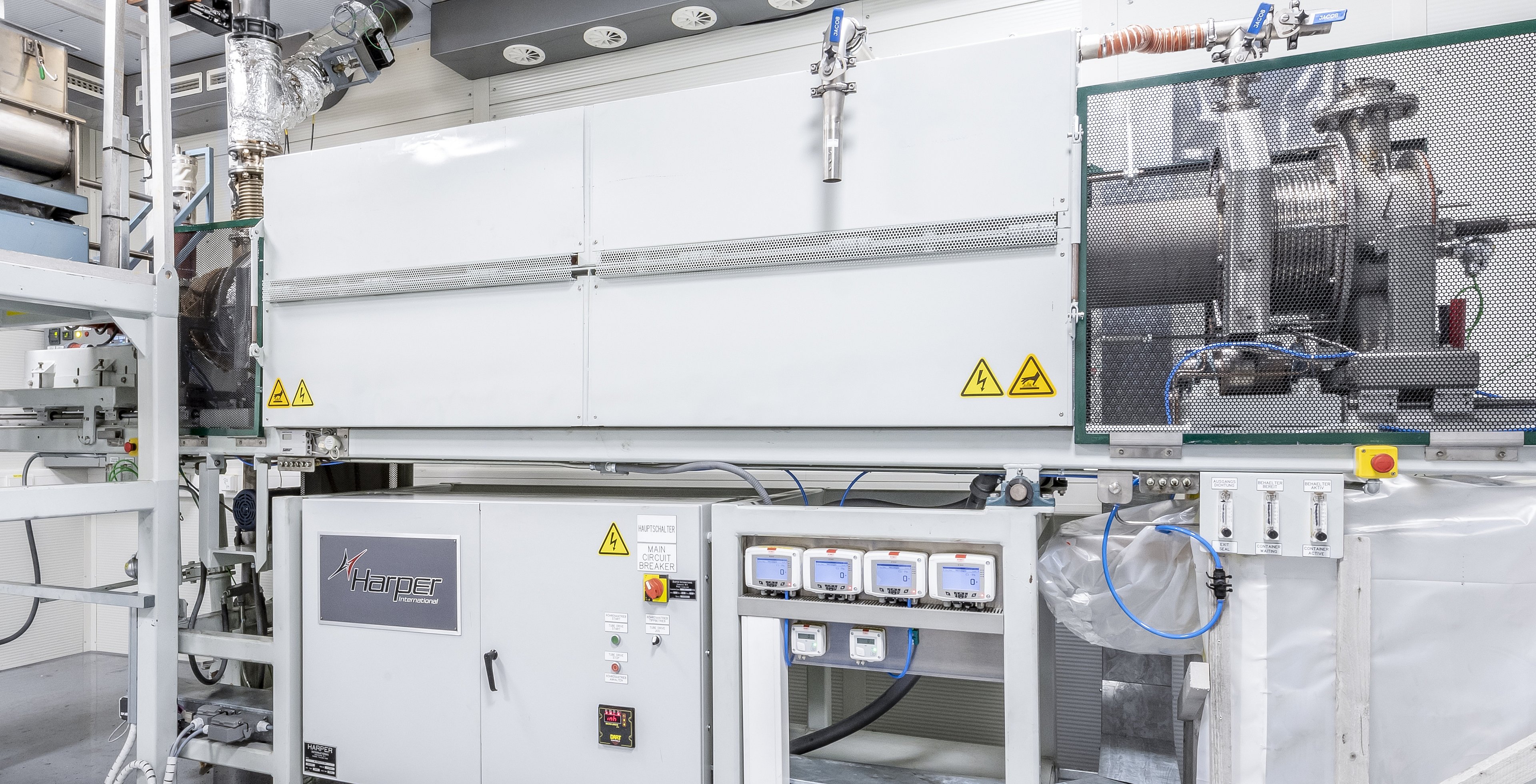
Sodium-Ion Batteries (NIB)
The most commonly used electrochemical energy storage technology for stationary, portable and automotive applications is the lithium-ion battery (LIB). However, due to the limited availability of lithium, the high demand leads to challenges and risks. In addition, there are increasing supply risks due to (geo-)political causes. The currently intensively researched sodium-ion technology offers a future alternative to the LIB.
Contact us
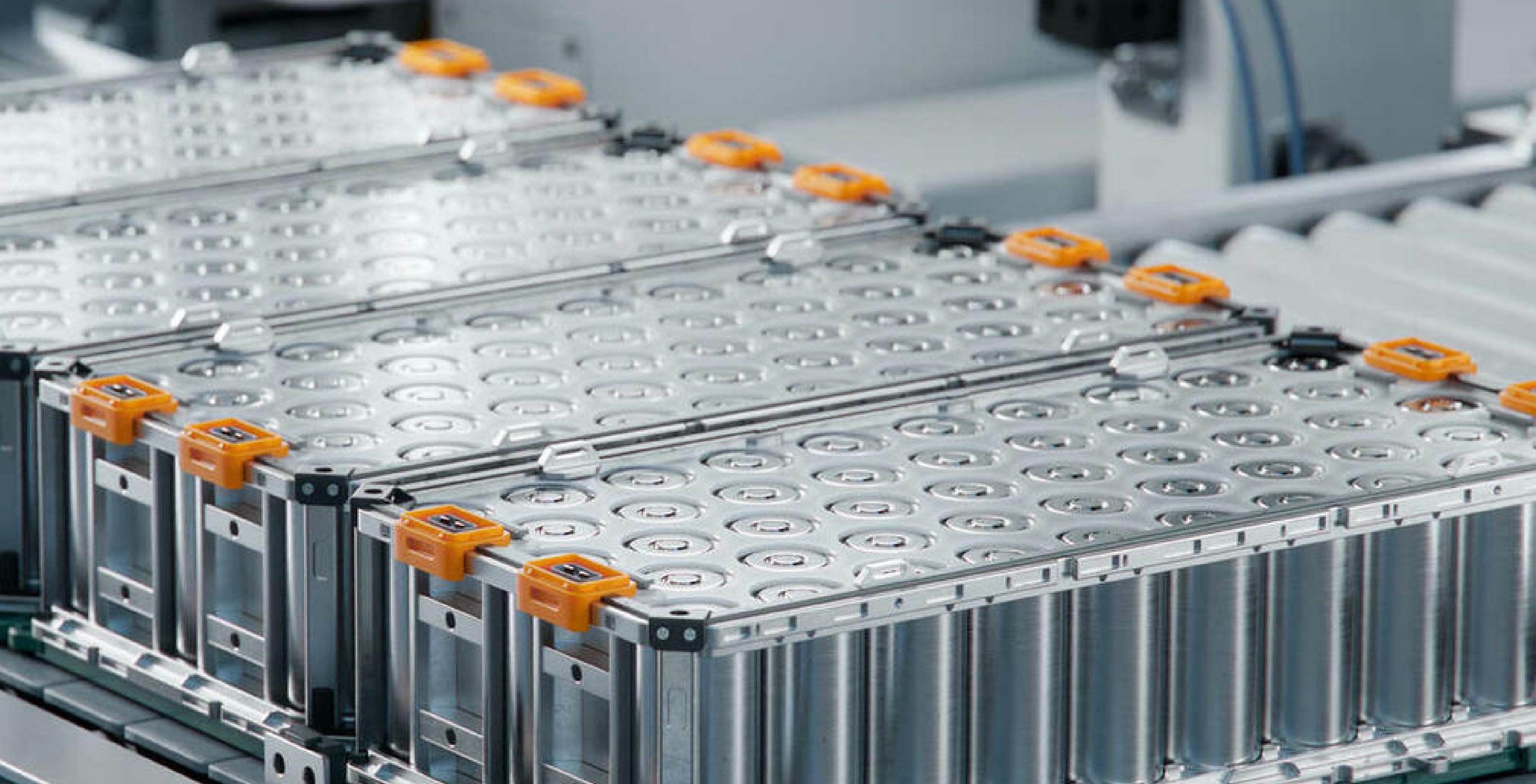
Layered Sodium Oxides
Sodium layered oxides represent a class of cathode materials for sodium-ion batteries. The material properties can be influenced by the choice of transition metals and the sodium content. In addition, a good balance of structural and electrochemical electrode properties can be achieved by combining different metals.
Advantages and Disadvantages of Sodium-Ion Batteries
Compared to lithium analogs, sodium layered oxides offer greater chemical diversity due to structural differences. While only the critical and costly metals cobalt and nickel are possible with lithium layered oxides, less critical elements such as titanium and iron can be used with sodium. In addition, the better global availability of sodium offers a significant cost advantage. Further cost savings result from the possibility of using aluminum as a current collector for both electrodes. The combination of savings opportunities offer a price advantage of approximately 16% over current LFP cells in perspective. In application, NIBs have the advantage that they can be charged and discharged at lower temperatures. This results in cost advantages, as heating and cooling systems can largely be dispensed with at module and system level. Furthermore, higher discharge rates can be realized in continuous operation. Due to the possibility of transporting Na-ion batteries in a deep-discharged state (0V), there are advantages in handling as well as transport costs, since there is no need to classify dangerous goods. In the area of cell production, a "drop-in" solution is possible, which can lead to high market penetration for EES and "low-cost" mobility solutions. Existing production facilities of LIBs can be used for NIBs, which makes production-side implementation possible quickly without immense additional costs. .
One disadvantage is the currently comparatively short service life due to the strong structural changes during charging and discharging. However, the most significant disadvantage compared to LIBs is the lower energy density, due to the size and higher weight of the sodium ions. Due to the intrinsic gravimetric energy density, NIBs are particularly suitable for use in the electromobility sector for short distances, frequent charging or stationary storage.